This content is limited to our registered members
-
Access exclusive business services unlimited
-
Access valuable features : audio listening & tools sharing with your patients
-
Access more than 150 product sheets, dedicated to professionals
You already have an account ? login now
Updates on dermatology: Proteome Science
Summary of the Symposium held during the IMCAS World Congress in Paris in January 2023: “A fundamental discovery on aging: beyond the genome, the proteome”, with Dr. Marco Rocha, Prof. Miroslav Radman, and Isabelle Benoit (Naos).
Related topics

Stéphane Fauverghe
NAOS International Medical Relations Director
Dear all,
I am very pleased to present you the fifth edition of the Bioderma/Naos Updates on Dermatology.
Bioderma/Naos has been regularly organizing international events dedicated to Dermatology. These events, for dermatologists and all healthcare professionals interested in Dermatology, are always presented by renowned experts in their field. In our approach to promote the development of knowledge in Dermatology, we have the pleasure to offer you this new publication. You will find hereafter the summary of the Naos Symposium held during the IMCAS World Congress in Paris in January 2023 - A fundamental discovery on aging: beyond the genome, the proteome. The speakers were Marco Rocha from Brazil, Miroslav Radman from Croatia, and Isabelle Benoit from France (Naos).
During this symposium, Miroslav Radman presented a proteome centric view of aging and age-related diseases. Isabelle Benoit delivered a lecture on the proteome alteration as the root-cause of skin aging. And Marco Rocha presented the protection of the proteome as a new therapeutical path for healthy aging.
I wish you all an enjoyable, enriching, and interesting reading
- Speakers’s biographies
- Fundamental common mechanism of aging and age-related disease - Miroslav Radman (Split, Croatia)
- A change of paradigm for a new scientific era - Isabelle Benoit (Aix-en-Provence, France)
- Protection of the proteome: a new therapeutic way for healthy aging - Marco Rocha (SĂŁo Paulo, Brazil)

MedILS, Split Croatia - INSERM unit 1001, University R. Descartes, Paris, France
What do the following three questions have in common?
- What does time do to cells and organisms to cause morbidity and mortality?
- What determines species-specific longevity?
- What determines the predispositions to different age-related diseases (ARD)? The answer is: oxidative damage to the proteome.
Aging and age-related diseases (ARD) share a common biological clock that appears to be their root cause: protein damage. Two variables appear common in the studies of the aging process of all living organisms: Reactive Oxygen Species (ROS) and disturbed tissue homeostasis. Figure 1 shows the shared variables from the studies of aging in invertebrate model organisms, mammals and human progerias.(1)
Currently, it is recognized that virtually every diseased state involves some degree of inflammation and oxidative stress. In normal, healthy tissue, redox chemistry and ROS are kept under control to maintain homeostasis at the cellular level.(2) Cellular generation of ROS occurs from both enzymatic and non-enzymatic processes.(3, 4) However, ROS can also be the outcome of acute cell stress and may cause cell death via apoptosis or necrosis.
Proteins are major biological targets of ROS and oxidative stress when these are formed in vivo, either in intra- or extracellular compartments. Proteins are able to scavenge 50 to 75% of free ROS such as -OH within a cell by g-radiolysis.(5, 6) Figure 2 shows how ROS inflicts protein damage in cells and organisms.
Some ROS-induced protein modifications have a preference to occur in misfolded proteins and then provoke further unfolding of the protein structure, while some are essentially harmless events.(7)
Irreversible protein modifications and aggregates lead to the inactivation of both protein functions and interactions with other proteins or molecules, resulting in harmful cellular effects and diseases.(8) Among the consequences of oxidative damage to proteins are cell dysfunction, aging and diseases as shown in Figure 3.
However, this process can be reversible. In embryonic stem cells and in Deinococcus radiodurans, the ratio of carbonylated and intact proteins is in favour of the latter.
In young, healthy organisms, the concentration of ROS and misfolded proteins is balanced, and both are present in small quantities. Oxidative damage targets misfolded proteins, resulting in an increased level of irreversibly misfolded proteins. Once oxidatively damaged proteins reach a certain level, premature death - mainly by ARD - occurs by the catastrophic loss of protein quality control.(9)
Cellular life is maintained by protein activities; therefore protein damage ruins the functions of life, including the prevention of molecular damage and repair of damaged DNA, proteins and other damaged cellular components.
Hence, cellular fitness decreases due to persisting protein damage, leading to the progressive degeneration of cellular functions and even direct cell death, due to the cytotoxic structures of damaged proteins (Figure 4). The ultimate liability to protein function is an irreversible oxidative protein modification called protein carbonylation (PC).(10-12) Since cellular aging can be defined as a progressive degeneration of vital cellular functions, and an increase in mutation rates, oxidative proteome damage appears as the most likely cause of aging and ARD, including cancer.(10)
In conclusion, oxidative damage to the proteome can be considered as the root cause of aging and all, or nearly all, ARD.
Figure 1
Pathways shared between aging in invertebrate model organisms, mammals and human progerias
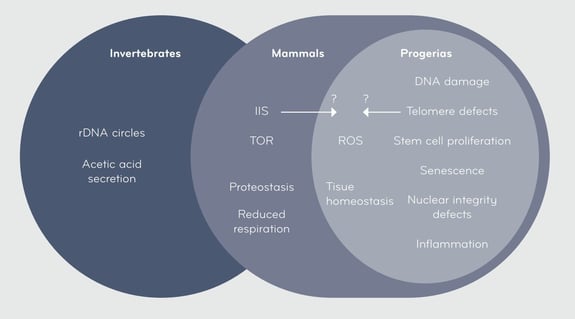
Figure 2
Impact of reactive oxygen species on the organism
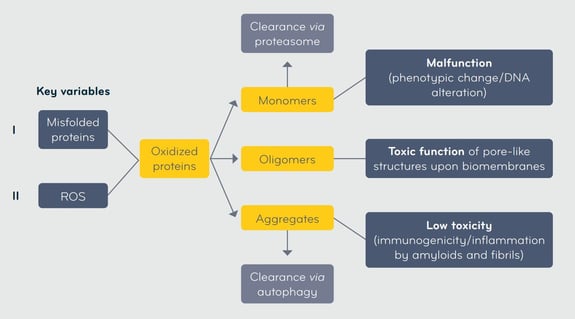
Figure 3
Oxidation process resulting in cell dysfunction, aging and diseases
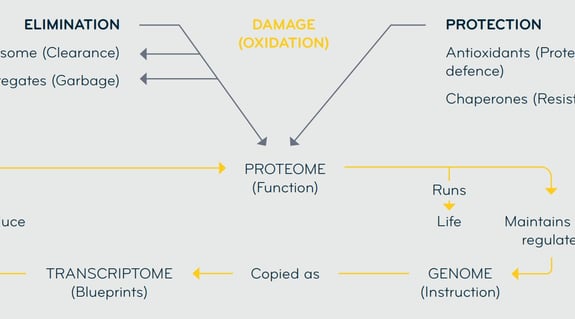
Figure 4
Essentials of aging and diseases
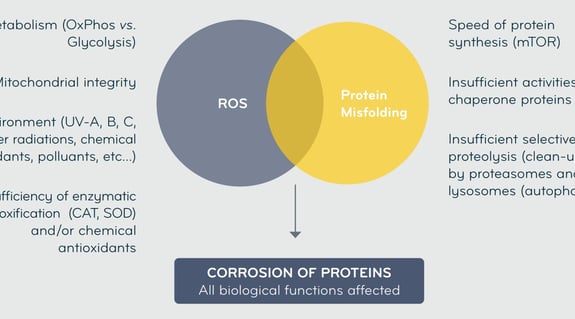
Conclusion
In conclusion, oxidative damage to the proteome can be considered as the root cause of aging and all, or nearly all, agerelated diseases.
References
- Burtner CR, Kennedy BK. Progeria syndromes and aging: what is the connection? Nature reviews Molecular cell biology. 2010;11(8):567-78.
- Hensley K, Floyd RA. Reactive oxygen species and protein oxidation in aging: a look back, a look ahead. Archives of biochemistry and biophysics. 2002;397(2):377-83.
- Thannickal VJ, Fanburg BL. Reactive oxygen species in cell signaling. Am J Physiol Lung Cell Mol Physiol. 2000;279(6):L1005-28.
- Moldovan L, Moldovan NI. Oxygen free radicals and redox biology of organelles. Histochemistry and cell biology. 2004;122(4):395-412.
- Davies MJ, Fu S, Wang H, Dean RT. Stable markers of oxidant damage to proteins and their application in the study of human disease. Free radical biology & medicine. 1999;27(11-12):1151-63.
- Gebicki JM. Protein hydroperoxides as new reactive oxygen species. Redox report : communications in free radical research. 1997;3(2):99-110.
- Cabiscol E, Ros J. Oxidative Damage to Proteins: Structural Modifications and Consequences in Cell Function. 2006. p. 399-471.
- Dalle-Donne I, Aldini G, Carini M, Colombo R, Rossi R, Milzani A. Protein carbonylation, cellular dysfunction, and disease progression. Journal of cellular and molecular medicine. 2006;10(2):389-406.
- Schneider K, Bertolotti A. Surviving protein quality control catastrophes- -from cells to organisms. Journal of cell science. 2015;128(21):3861-9.
- Krisko A, Radman M. Biology of extreme radiation resistance: the way of Deinococcus radiodurans. Cold Spring Harbor perspectives in biology. 2013;5(7).
- Krisko A, Radman M. Protein damage and death by radiation in Escherichia coli and Deinococcus radiodurans. Proceedings of the National Academy of Sciences of the United States of America. 2010;107(32):14373-7.
- Oliver CN, Ahn BW, Moerman EJ, Goldstein S, Stadtman ER. Age-related changes in oxidized proteins. J Biol Chem. 1987;262(12):5488-91.

NAOS-ILS, Aix-en-Provence, France
Extremophilic organisms are considered as models of biological robustness to aging.
Research conducted by KRISKO and RADMAN showed that the extremophilic bacterium Deinococcus radiodurans is able to fix DNA damage using non-oxidable repairing proteins, thus being almost eternal.(1) Results also confirmed that longevity is not exclusively linked to the genetic code, but mainly to the capacity of the organism to repair this code rapidly; the authors concluded that protecting the tool that repairs and protects the DNA, i.e. the proteome, which comprises all proteins of the body, may be key for cellular longevity. Thus, a damaged proteome or the loss of proteostasis may be considered as the first step, the root cause, of cellular dysfunction, leading to diseases and aging.
With 15 to 27.5% of its total composition, proteins are the second main component of the skin after water.(2) Proteins such as collagen, elastin, keratin, and many more elements that constitute it play a structural role in the skin. Functionally, proteins are involved in cellular metabolism and tissue homeostasis through enzymes, cytokines, hormones and growth factors, as well as other functional elements. Damaging the skin proteome may impair cellular and tissular functioning, thereby causing skin alteration and skin aging. Thus, protecting the proteome may be essential for skin longevity and may be considered as the meta theory beyond most currently accepted theories of aging.
LĂłpez-OtĂn et al. proposed the Biological Geriatric Assessment (BGA) concept.(3) This concept describes nine hallmarks that are currently considered as the denominators of body aging. BGA includes genomic instability, telomere attrition, epigenetic alterations, dysregulated nutrient sensing, mitochondrial dysfunction, cellular senescence, chronic inflammation, stem cell exhaustion, altered intercellular communication and loss of proteostasis (protein homeostasis).(4) Today, telomer attrition and chronic inflammation are the most frequently discussed mechanisms in aging(5, 6) and can be related to proteome damage. Thus protecting the proteome encompasses preventing telomers attrition and chronic inflammation.
Several alterations of numerous proteins have been reported in tissue aging. Such proteins are highly sensitive and impacted by Reactive Oxygen Species (ROS).(7, 8) During aging, senescent cells accumulate in all tissues and contribute to their functional decline via the Senescence-Associated Secretory Phenotype (SASP).(9) SASP is thought to trigger several pathological aging features, among them chronic inflammation, which is the principal biomarker of aging.(10) Sirtuins are protein deacetylases associated with aging. They are involved in chronic inflammation through the inactivation of NLRP3, which inhibits aging.(7, 11) Cell Senescence (CS) is associated with telomere shortening, which leads to permanent cell cycle arrest.(12) Consequently, preventing telomere attrition by protecting their protein coat may thus also prevent CS and related chronic inflammation in tissues.(13)
The accumulation of carbonylated proteins has been associated with skin aging.(14, 15) Protein Carbonylation (PC) is irreversible oxidative damage, frequently resulting in the loss of protein function and aggregation.(16) In the skin, PC results in distinguishable clinical changes. Transepidermal water loss is increased in the stratum corneum.(17) In the supra-basal epidermis, the accumulation of carbonylated keratins disrupts light transmission, which, in turn, alters the subjectively-perceived skin radiance and homogeneity of skin complexion.(18, 19) Carbonylated proteins alter the dermis by damaging collagen and elastin, and by altering fibroblasts and causing changes in the expression of metalloproteases, such as MMP-1, resulting in chronic inflammation involving IL-8.(20, 21) Therefore, preventing PC and maintaining the balance between protein synthesis and degradation is essential in maintaining a youthful appearance of the skin, reflecting a harmonious cellular biochemistry.
Protein Quality Control (PQC), involving multiple chaperones and degradative pathways, identifies and eliminates abnormal and misfolded proteins that are deleterious to cells and tissues.(22) If PQC fails, then protein aggregation may occur. While many of these aggregations are eliminated via autophagy, others accumulate in the cells and tissues, leading to cell damage and apoptosis and may be considered markers for aging and age-related diseases.(23-25)
Until recently, much research about aging has focused on the protection and repair of DNA, as well as genes that control and prevent cell and tissue aging. However, since proteins repair DNA and synthesize antioxidant protecting molecules, it is not surprising that, for instance, extreme radiation resistance is achieved by the protection of the proteome, rather than the genome.(26) Therefore, protecting the proteome may prevent the body from signs of aging through chaperone systems that stabilize the conformation of proteins and antioxidant molecules that neutralize carbonylation triggers.
Recent research has shown that antioxidant chaperones constitute a major breakthrough compared to current antioxidant approaches in this deleterious process.
Arthrobacter agilis (A. agilis), an extremophilic bacterium isolated from snow crystals is ultra-resistant to UV radiation, very low temperatures and oxidative stress. It is able to self-repair and survive in extreme conditions.(27)
The NAOS-patented extract of A. agilis is a synergistic combination of 6 anti-oxidant chaperone-like molecules, also called bacterioruberins. They are unique in nature and have a distinctive affinity for proteins, providing a protective, chaperone-like activity, as well as a powerful antioxidant effect. An in vitro heat test, which uses heat to destabilize protein alkaline phosphatase, showed that A. agilis extract provides protection of more than 80% of the activity of the 3-D protein structure compared to currently available anti-oxidants. This highly protective capacity is due to its specific chaperone-like activity (Figure 1). Other work has confirmed the high antioxidant potential of A. agilis extract compared to different reference molecules (Figure 2). Moreover, in vitro investigations have demonstrated that the A. agilis extract protects elastin from oxidative stress (Figure 3) induced by UVA radiation and pollution (Figure 4), and that it physically protects DNA repair enzymes (T4 endonuclease) and their functionality against UVA irradiation (Figure 5). Moreover, it significantly shields cultured keratinocytes against carbonylation induced by UV and blue light radiation, as well as pollution (Figure 6).
Figure 1
Heat test confirming in vitro the high protective potential of Arthrobacter agilis extract of the 3-D protein structure compared to reference molecules
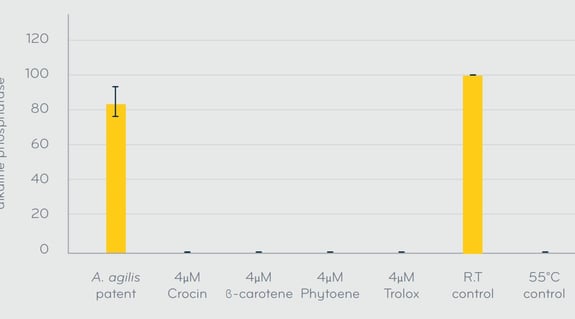
Figure 2
In vitro antioxidant potential of Arthrobacter agilis extract compared to reference antioxidants
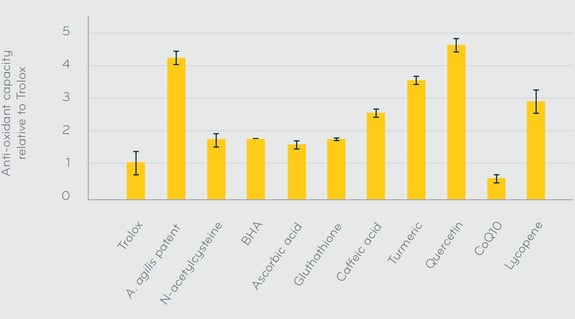
Figure 3
Protective potential of Arthrobacter agilis extract against oxidative stress of elastin compared to α-tocopherol
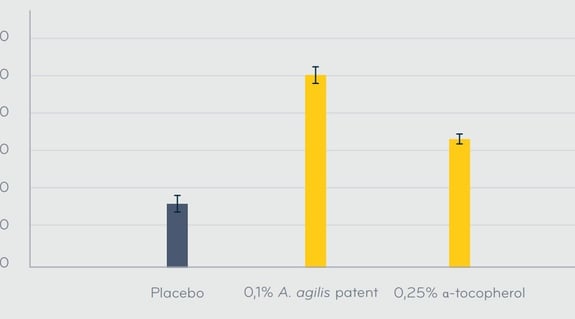
Figure 4
Protective potential in skin explants of Arthrobacter agilis extract against UV radiation and pollution compared to α-tocopherol
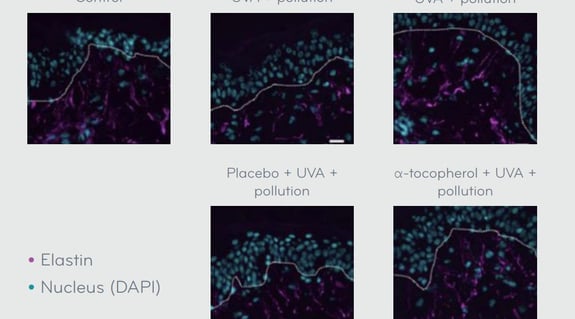
Figure 5
In vitro testing of the functional protective potential of Arthrobacter agilis extract of T4 endonuclease against UVA irradiation
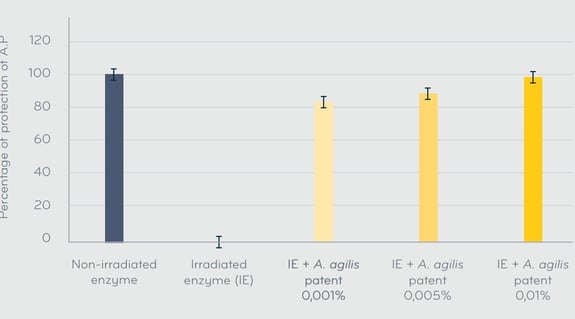
Conclusion
In conclusion, a proteomics-centered R&D strategy consisting in protecting the proteome rather than the genome opens the perspective of major breakthroughs in age management. Protecting the proteome is the meta theory beyond most other theories about aging. Using antioxidant molecules with a chaperone-like activity, such as bacterioruberins instead of “classical” antioxidants, is a novel and promising approach to protect the proteome and to impact aging, including that of the skin.
References
- Krisko A, Radman M. Protein damage and death by radiation in Escherichia coli and Deinococcus radiodurans. Proceedings of the National Academy of Sciences of the United States of America. 2010;107(32):14373-7.
- Wang Z, Shen W, Kotler DP, Heshka S, Wielopolski L, Aloia JF, et al. Total body protein: a new cellular level mass and distribution prediction model. The American journal of clinical nutrition. 2003;78(5):979-84.
- LĂłpez-OtĂn C, Blasco MA, Partridge L, Serrano M, Kroemer G. The Hallmarks of Aging. Cell. 2013;153(6):1194-217.
- Ellis KJ. Human body composition: in vivo methods. Physiological reviews. 2000;80(2):649-80.
- Coppé JP, Patil CK, Rodier F, Krtolica A, Beauséjour CM, Parrinello S, et al. A human-like senescence-associated secretory phenotype is conserved in mouse cells dependent on physiological oxygen. PLoS One. 2010;5(2):e9188.
- Kuilman T, Michaloglou C, Vredeveld LC, Douma S, van Doorn R, Desmet CJ, et al. Oncogene-induced senescence relayed by an interleukin-dependent inflammatory network. Cell. 2008;133(6):1019-31.
- Gritsenko A, Green JP, Brough D, Lopez-Castejon G. Mechanisms of NLRP3 priming in inflammaging and age related diseases. Cytokine Growth Factor Rev. 2020;55:15-25.
- Ma J, Liu M, Wang Y, Xin C, Zhang H, Chen S, et al. Quantitative proteomics analysis of young and elderly skin with DIA mass spectrometry reveals new skin aging-related proteins. Aging. 2020;12(13):13529-54.
- Fitsiou E, Pulido T, Campisi J, Alimirah F, Demaria M. Cellular Senescence and the Senescence-Associated Secretory Phenotype as Drivers of Skin Photoaging. Journal of Investigative Dermatology. 2021;141 (4, Supplement):1119-26.
- Neves J, Demaria M, Campisi J, Jasper H. Of flies, mice, and men: evolutionarily conserved tissue damage responses and aging. Dev Cell. 2015;32(1):9-18.
- He M, Chiang HH, Luo H, Zheng Z, Qiao Q, Wang L, et al. An Acetylation Switch of the NLRP3 Inflammasome Regulates Aging-Associated Chronic Inflammation and Insulin Resistance. Cell metabolism. 2020;31(3):580-91.e5.
- Di Micco R, Krizhanovsky V, Baker D, d'Adda di Fagagna F. Cellular senescence in aging: from mechanisms to therapeutic opportunities. Nat Rev Mol Cell Biol. 2021;22(2):75-95.
- De Graff AM, Hazoglou MJ, Dill KA. Highly Charged Proteins: The Achilles' Heel of Aging Proteomes. Structure (London, England : 1993). 2016;24(2):329-36.
- Wagner KH, Cameron-Smith D, Wessner B, Franzke B. Biomarkers of Aging: From Function to Molecular Biology. Nutrients. 2016;8(6).
- Cabiscol E, Tamarit J, Ros J. Protein carbonylation: proteomics, specificity and relevance to aging. Mass Spectrom Rev. 2014;33(1):21-48.
- Dalle-Donne I, Aldini G, Carini M, Colombo R, Rossi R, Milzani A. Protein carbonylation, cellular dysfunction, and disease progression. Journal of cellular and molecular medicine. 2006;10(2):389-406.
- Iwai I, Hirao T. Protein carbonyls damage the water-holding capacity of the stratum corneum. Skin pharmacology and physiology. 2008;21(5):269-73.
- Thiele JJ, Hsieh SN, Briviba K, Sies H. Protein oxidation in human stratum corneum: susceptibility of keratins to oxidation in vitro and presence of a keratin oxidation gradient in vivo. J Invest Dermatol. 1999;113(3):335-9.
- Iwai I, Ikuta K, Murayama K, Hirao T. Change in optical properties of stratum corneum induced by protein carbonylation in vitro. Int J Cosmet Sci. 2008;30(1):41-6.
- Bonté F, Girard D, Archambault JC, Desmoulière A. Skin Changes During Aging. Subcell Biochem. 2019;91:249-80.
- Yamawaki Y, Mizutani T, Okano Y, Masaki H. The impact of carbonylated proteins on the skin and potential agents to block their effects. Exp Dermatol. 2019;28 Suppl 1:32-7.
- Hanna J, Guerra-Moreno A, Ang J, Micoogullari Y. Protein Degradation and the Pathologic Basis of Disease. The American journal of pathology. 2019;189(1):94-103.
- Hipp MS, Kasturi P, Hartl FU. The proteostasis network and its decline in aging. Nat Rev Mol Cell Biol. 2019;20(7):421-35.
- Ravindran MS. Molecular chaperones: from proteostasis to pathogenesis. Febs j. 2018;285(18):3353-61.
- Budenholzer L, Cheng CL, Li Y, Hochstrasser M. Proteasome Structure and Assembly. Journal of molecular biology. 2017;429(22):3500-24.
- Krisko A, Radman M. Protein damage, aging and age-related diseases. Open Biol. 2019;9(3):180249.
- Fong N, Burgess M, Barrow K, Glenn D. Carotenoid accumulation in the psy chrotrophic bacterium Arthrobacter agilis in response to thermal and salt stress. Applied microbiology and biotechnology. 2001;56:750-6.

Unifesp, SĂŁo Paulo, Brazil
The human body is composed of about 60% water, 16% proteins, as well as fats and other components.(1) The human genome is made of about 20,000 to 25,000 genes and alternative promoters, splicing and mRNA. Editing processes lead to the transcriptome of about 100,000 transcripts which, through post-translational modifications processes, produce more than 1,000,000 different proteins.(2)
The proteome comprises the ensemble of proteins in any organism.(3) Proteins are the most diverse and structurally complex macromolecules in the cell. They participate in nearly every known aspect of life, either directly or by synthesizing other active biomolecules. Their function is determined by specific native three-dimensional structures. These structures ensure that new polypeptide chains adopt, and native proteins keep, their properly folded conformation, even during stressful situations.(4, 5)
A healthy proteome is achieved and maintained by proteostasis, an optimal turn-over process of proteins, with a high ribosomal fidelity in translation and a high accuracy of protein folding assisted by Chaperone Proteins (CP). This process is also called Protein Quality Control (PQC) via the proteostasis network.(6, 7) Failing of PQC results in a low quality proteome that misfunctions or leads to detrimental accumulation of misfolded proteins that form toxic small aggregates and large amyloids or fibrils.(8) It has been shown that protein oxidation precedes aggregation, to the extent that over 90% of carbonylated proteins are found in aggregates.(9) This is explained by a high sensitivity to the oxidation of misfolded proteins.(10)
In the skin, protein damage mostly results from external stimuli, including exposure to oxidants present in air pollution, ozone, chemical agents and radiation, such as UV light.(11-16) Moreover, protein damage can be caused through internally generated oxidants and reactive oxygen and nitrogen species, which are produced during metabolism or immune responses.(17, 18) Finally, they can be produced because of transcriptional or translational errors, since misfolded proteins become sensitive to carbonylation.(19, 20) The resulting modified cornified envelope leads to an altered anti-oxidative capacity and the reduced barrier function of the epidermis.(21)
Oxidative stress plays a major role in skin disease and the aging process. It is the imbalance between pro- and antioxidants in favor of the former, causing proteotoxicity.(22) Proteotoxicity is cellular dysfunction, caused by protein misfolding, damage and aggregation.
It is used as a biomarker for a number of Age-Related Degeneration and Diseases (ARDD), including psoriasis, Atopic Dermatitis (AD) and cancers, as well as aging.(23, 24)
In psoriasis, the multifunctional cytokine Tumor Necrosis Factor-alpha (TNF-alpha) plays an important role in the inflammatory and immunological response of the skin. Reactive ROS are involved in the TNF-alpha-induced signaling pathways, leading to the production of primary human keratinocytes inducing the release of cytokines, inflammation and Protein Carbonylation (PC).(25)
In AD, PC may play a significant role. The etiopathogenesis of AD is complex and multifactorial, with a mix of genetic, immunological and environmental aspects, and its physiopathology is still not completely elucidated. However, as in other chronic inflammatory diseases, oxidative stress may play an important pathogenetic role.(26)
In skin cancer, growing evidence suggests that the efficiency of DNA repair after exposure to UV radiation is highly dependent on the levels of oxidative protein damage, including, but not limited to, DNA repair proteins. Besides DNA lesions, UV-induced oxidative stress can indeed result in the carbonylation of proteins, a major form of irreversible protein damage that inactivates their biological function.(27)
Nine hallmarks are currently considered as the denominators of aging, including that of the skin.(28) They include genomic instability, telomere attrition, epigenetic alterations, dysregulated nutrient sensing, mitochondrial dysfunction, cellular senescence, chronic inflammation, stem cell exhaustion, altered intercellular communication and loss of proteostasis or protein homeostasis.
Skin and tissue aging via protein damage is mainly caused by protein glycation, carbonylation and, to a lesser extent, through carbamylation. The accumulation of Advanced Glycation End products (AGEs), protein carbamylation and PC have been causally associated with skin aging.(29-31) PC forms reactive ketones or aldehydes.(32)
In the skin, PC results in three distinguishable clinical changes, as presented by Isabelle Benoît. Transepidermal water loss is increased in the stratum corneum.(33-35) In the supra-basal epidermis, the accumulation of carbonylated keratins disrupts light transmission that alters the subjectively perceived skin radiance and homogeneity of skin complexion.(36, 37) Carbonylated proteins alter the dermis by degrading collagen and elastin. PC is associated with an alteration of fibroblasts, as well as changes in the expression of metalloproteases such as MMP-1, the development of a chronic inflammation involving IL-8.(38-42) In the skin, as in any other tissue, a damaged proteome may be considered as the first step, the root cause, of cellular dysfunction, leading to diseases and aging.
There is growing evidence that protecting the proteome will help to build and maintain healthy tissues and thus allow to slow down the process of aging, in particular skin aging.
References
- Wang Z, Shen W, Kotler DP, Heshka S, Wielopolski L, Aloia JF, et al. Total body protein: a new cellular level mass and distribution prediction model. Am J Clin Nutr. 2003;78(5):979-84.
- Wysocki VH, Resing KA, Zhang Q, Cheng G. Mass spectrometry of peptides and proteins. Methods. 2005;35(3):211-22.
- 2D Electrophoresis: From Protein Maps to Genomes. Proceedings of the International Meeting. Siena, Italy, September 5-7, 1994. Electrophoresis. 1995;16(7):1077-322.
- Balchin D, Hayer-Hartl M, Hartl FU. In vivo aspects of protein folding and quality control. Science (New York, NY). 2016;353(6294):aac4354.
- Bukau B, Weissman J, Horwich A. Molecular chaperones and protein quality control. Cell. 2006;125(3):443-51.
- Hipp MS, Kasturi P, Hartl FU. The proteostasis network and its decline in aging. Nat Rev Mol Cell Biol. 2019;20(7):421-35.
- Ravindran MS. Molecular chaperones: from proteostasis to pathogenesis. The FEBS journal. 2018;285(18):3353-61.
- Kaushik S, Cuervo AM. Proteostasis and aging. Nat Med. 2015;21(12):1406-15.
- Tanase M, Urbanska AM, Zolla V, Clement CC, Huang L, Morozova K, et al. Role of Carbonyl Modifications on Aging-Associated Protein Aggregation. Sci Rep. 2016;6:19311.
- Krisko A, Radman M. Protein damage, aging and age-related diseases. Open Biol. 2019;9(3):180249.
- Ritz B, Hoffmann B, Peters A. The Effects of Fine Dust, Ozone, and Nitrogen Dioxide on Health. DeutArztblInt. 2019;51-52(51-52):881-6.
- Mirowsky JE, Dailey LA, Devlin RB. Differential expression of pro-inflammatory and oxidative stress mediators induced by nitrogen dioxide and ozone in primary human bronchial epithelial cells. InhalToxico l. 2016;28(8):374-82.
- Murray AR, Kisin E, Castranova V, Kommineni C, Gunther MR, Shvedova AA. Phenol-induced in vivo oxidative stress in skin: evidence for enhanced free radical generation, thiol oxidation, and antioxidant depletion. ChemResToxicol. 2007;20(12):1769-77.
- McDaniel D, Farris P, Valacchi G. Atmospheric skin aging-Contributors and inhibitors. J Cosmet Dermatol. 2018;17(2):124-37.
- Mohania D, Chandel S, Kumar P, Verma V, Digvijay K, Tripathi D, et al. Ultraviolet Radiations: Skin Defense-Damage Mechanism. Adv Exp Med Biol. 2017;996:71-87.
- Kalim S. Protein carbamylation in end stage renal disease: is there a mortality effect? Curr Opin Nephrol Hypertens. 2018;27(6):454-62.
- Baeuerle PA, Rupec RA, Pahl HL. Reactive oxygen intermediates as second messengers of a general pathogen response. Pathol Biol. 1996;44(1):29-35.
- Valko M, Jomova K, Rhodes CJ, KuÄŤa K, MusĂlek K. Redox- and non-redox-metal-induced formation of free radicals and their role in human disease. Arch Toxicol. 2016;90(1):1-37.
- Dukan S, Farewell A, Ballesteros M, Taddei F, Radman M, Nyström T. Protein oxidation in response to increased transcriptional or translational errors. Proc Natl Acad Sci USA. 2000;97(11):5746-9.
- Krisko A, Radman M. Phenotypic and genetic consequences of protein damage. PLoS Genet. 2013;9(9):e1003810.
- Rinnerthaler M, Bischof J, Streubel MK, Trost A, Richter K. Oxidative stress in aging human skin. Biomolecules. 2015;5(2):545-89.
- Giustarini D, Dalle-Donne I, Tsikas D, Rossi R. Oxidative stress and human diseases: Origin, link, measurement, mechanisms, and biomarkers. Crit Rev Clin Lab Sci. 2009;46(5-6):241-81.
- Ruz C, Alcantud JL, Vives Montero F, Duran R, Bandres-Ciga S. Proteotoxicity and Neurodegenerative Diseases. Int J Mol Sci.2020;21(16):5646.
- Dalle-Donne I, Giustarini D, Colombo R, Rossi R, Milzani A. Protein carbonylation in human diseases. Trends Mol Sci. 2003;9(4):169-76.
- Xu J, Chen H, Qian H, Wang F, Xu Y. Advances in the modulation of ROS and transdermal administration for anti-psoriatic nanotherapies. J Nano Bio. 2022;20(1):448.
- Bertino L, Guarneri F, Cannavò SP, Casciaro M, Pioggia G, Gangemi S. Oxidative Stress and Atopic Dermatitis. Antioxidants (Basel, Switzerland). 2020;9(3).
- Tramutola A, Falcucci S, Brocco U, Triani F, Lanzillotta C, Donati M, et al. Protein Oxidative Damage in UV-Related Skin Cancer and Dysplastic Lesions Contributes to Neoplastic Promotion and Progression. Cancers. 2020;12(1):110.
- LĂłpez-OtĂn C, Blasco MA, Partridge L, Serrano M, Kroemer G. The Hallmarks of Aging. Cell. 2013;153(6):1194-217.
- Wagner KH, Cameron-Smith D, Wessner B, Franzke B. Biomarkers of Aging: From Function to Molecular Biology. Nutrients. 2016;8(6).
- Cabiscol E, Tamarit J, Ros J. Protein carbonylation: proteomics, specificity and relevance to aging. Mass Spectrom Rev. 2014;33(1):21-48.
- Jaisson S, Pietrement C, Gillery P. Protein Carbamylation: Chemistry, Pathophysiological Involvement, and Biomarkers. Adv Clin Chem Ad. 2018;84:1-38.
- Suzuki YJ, Carini M, Butterfield DA. Protein carbonylation. Antioxid Redox Signal. 122010. p. 323-5.
- Tončić RJ, Kezić S, Hadžavdić SL, Marinović B. Skin barrier and dry skin in the mature patient. Clin Dermatol. 2018;36(2):109-15.
- Verdier-Sévrain S, Bonté F. Skin hydration: a review on its molecular mechanisms. J Cosm Dermatol. 2007;6(2):75-82.
- Iwai I, Hirao T. Protein carbonyls damage the water-holding capacity of the stratum corneum. Skin Pharmacol Physiol. 2008;21(5):269-73.
- Thiele JJ, Hsieh SN, Briviba K, Sies H. Protein oxidation in human stratum corneum: susceptibility of keratins to oxidation in vitro and presence of a keratin oxidation gradient in vivo. J Invest Dermatol. 1999;113(3):335-9.
- Iwai I, Ikuta K, Murayama K, Hirao T. Change in optical properties of stratum corneum induced by protein carbonylation in vitro. Int J Cosmet Sci. 2008;30(1):41-6.
- Bonté F, Girard D, Archambault JC, Desmoulière A. Skin Changes During Aging. Subcell Biochem. 2019;91:249-80.
- Bosset S, Barre P, Chalon A, Kurfurst R, Bonte F, Andre P, et al. Skin aging: clinical and histopathologic study of permanent and reducible wrinkles. Eur J Dermatol. 2002;12(3):247-52.
- Tigges J, Krutmann J, Fritsche E, Haendeler J, Schaal H, Fischer JW, et al. The hallmarks of fibroblast aging. Mech Aging Dev. 2014;138:26-44.
- Yamawaki Y, Mizutani T, Okano Y, Masaki H. The impact of carbonylated proteins on the skin and potential agents to block their effects. Exp Dermatol. 2019;28 Suppl 1:32-7.
- Moldogazieva NT, Mokhosoev IM, Mel'nikova TI, Porozov YB, Terentiev AA. Oxidative Stress and Advanced Lipoxidation and Glycation End Products (ALEs and AGEs) in Aging and Age-Related Diseases. Oxid Med Cell Long. 2019;2019:3085756.